Sci4Teens Competition: Age 13-15 Science Gold Winner
- cellfiemagazine
- Oct 11, 2020
- 9 min read
Potential Solutions to the Growing E-Waste Crisis - Kim Guo and Lindsay Xie
Abstract
In the past several years, the amount of e-waste generated has increased at an alarming rate, its impacts becoming much more severe regarding both the environment and human health. Accordingly, the consequences of the discarded plastic and metals are further worsened by the toxic substances in it. Similarly, chemicals and heavy metals are released during the production and recycling processes, heightening the already detrimental effects. A potential solution is investing in the recycling of e-waste, specifically biometallurgy, which has been proven to be one of the most sustainable recycling methods. With the addition of a plastic-eating bacterium called Ideonella sakaiensis, the amount of e-waste produced can be dramatically reduced. Despite the actions that can be taken by the community and electronics companies to minimize e-waste production and benefit the environment and economy, greater effort is needed to transition to a future with minimal e-waste.
E-Waste and Its Impacts
E-Waste consists of many factors that contribute to its growing concern regarding environmental pollution (see fig. 1). First, according to a United Nations report, 53.6 million tonnes of e-waste were dumped internationally in 2019 (Reuters). In the past five years, the amount of e-waste generated grew by 21%, while its recycling rate has decreased from 20% to 17.4% since 2017 (Loughran). Presently, 5G, the newest cellular network standard, is becoming more common, causing an increased requirement for phones supporting it. As the domestic waste stream is currently increasing at the quickest rate, the amount of discarded electronic devices would accelerate even more from this technological advancement (Semeuls). Moreover, some factors causing the rapid increase in electronic waste are companies’ marketing strategies and product packaging. One marketing strategy is planned obsolescence, which is where companies stop supporting OS updates after a certain amount of time or plan the expiry of their products’ batteries (“With E-Waste Posing”; Cho). The lifespans of computers and phones are also decreasing in order for companies to make more profit; in 1997, computers had an average lifespan of six years, but it decreased to two years in 2005 (Nimpuno et al. 33). In a society revolving around technological use, the change in lifespan produces an astounding level of waste. Additionally, companies providing electronics such as phones and laptops include accessories in the box. The chargers in these boxes generate about 300,000 tonnes of e-waste annually and are incredibly wasteful, especially as they are of low quality and are not used by consumers who already have multiple (Bohn). Furthermore, current recycling centres for e-waste could be harmful to nearby communities. With materials such as chemicals and different forms of plastic, these communities are contaminated by toxic pollutants released from recycling processes when extracting valuable substances. In all, the evolving technology industry causes the production of e-waste to increase rapidly, creating negative environmental impacts.
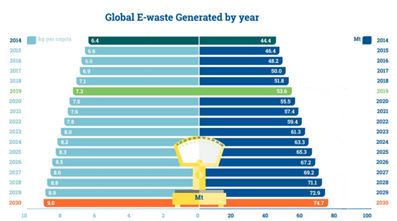
Fig. 1. Global E-waste Generated by year trend and prediction from Kirstin Linnenkoper; RecyclingInternational.com, 30 July 2020, https://recyclinginternational.com/e-scrap/un-reports-record-figures-for-e-waste/31123/.
Chemicals in E-Waste
Chemicals in Products
Electronic devices such as phones and computers consist of toxins that cause negative impacts on the environment and human health. Toxic chemicals present include brominated flame retardants, which are harmful to humans and the environment (“Brominated flame retardant”). Many of the metals in e-waste are carcinogenic and toxic; they consist of lead, cadmium, and beryllium. PVC plastic is also overly produced from electronics production; it consists of toxic substances such as phthalates and dioxins (Cho; “PVC: The Poison”).
Chemicals in Electronics Production
The production process of electronics releases many harmful chemicals that negatively affect workers and nearby regions. For example, some toxicants that workers are exposed to, including heavy metals and polybrominated diphenyl ethers, damage both the environment and human health; manufacturing facilities and neighbouring communities experience contaminated environments, with high chemical levels and deteriorating health (Nimpuno et al. 10-11). Likewise, the manufacturing processes of vital electronics components, such as semiconductors, printed circuit boards, and printing wiring assemblies, produce a significant amount of pollution. Some forms of air pollutants released are acids, toxic gases, and organic solvents; these could cause negative health impacts, especially when in contact. Forms of liquid waste, or effluents, include different acids, metals, and organic solvents; these substances pollute the drinking water of developing communities. The solid waste generated from electronics manufacturing could release more toxic chemicals into the atmosphere and contaminate the environment as well; examples include heavy metals, solder dross, and sludge (“Electronics Manufacturing” 302-303).
Chemicals in E-Waste Recycling
Both informal and formal e-waste recycling processes have many disadvantages that could be improved. In informal recycling centres, which are common in developing countries, the method of burning e-waste to extract valuable materials releases toxic chemicals such as carcinogenic dioxins. Burying e-waste is also performed frequently, causing toxins to leach into groundwater (“Harmful Effects Caused”). However, formal recycling centres are not less hazardous than informal recycling centres. They emit high levels of toxic chemicals such as diphenyl ethers and brominated flame retardants, making them dangerous to workers and nearby communities (Nimpuno et al. 11). Furthermore, hydrometallurgy, a formal recycling technique, uses aqueous solutions such as acids and cyanide to dissolve waste for metal recovery. This process is energy-consuming and harms human health, as it produces effluents that mix into aquatic ecosystems and drinking water (Kwok; Duan et al). Pyrometallurgy, a process that heats metals to high temperatures for purification, releases toxic gases that could cause environmental and health-related problems (Kwok; Arya and Kumar). Similar to hydrometallurgy, it is energy-consuming, making it neither cost-efficient nor eco-friendly (Awasthi et al). Overall, the chemicals generated in the recycling processes are highly disruptive to the environment.
Production and Recycling Processes
The Transparency of Electronics Companies
There has been a lack of reports regarding the use of heavy metals in specific electronics made by many companies due to their fear of design theft and stock value decrease. Consequently, products with devastating effects on their workers and the ecosystem will continue to be manufactured without proper precautions (González and Overeem). Likewise, due to the lack of transparency, e-waste recycling processes require extra steps to identify different metals, leading to lower efficiency.
Smart Packaging and Marketing
As newer electronics are produced and older ones increasingly become outdated, electronics companies are progressively becoming more accountable for the increased amount of e-waste. Many businesses are intentionally tweaking their product plans to gain more consumers and profit. For instance, several laptops require new cables, and various smartphone batteries are difficult to fix and expensive to replace. Similarly, older electronics are unable to be updated for new features and become unusable (Semeuls). Popular technology brands have recently been taking action to minimize e-waste. Notably, both Apple and Samsung plan to omit mobile phone accessories from their product boxes since most people already have excess charging cables and earphones (Peters). As chargers contribute 300,000 tonnes of e-waste annually, the quantity would decrease if accessories in device packages were eliminated (Bohn). Additional benefits of excluding cheap cables include fewer fossil fuels needed for powering ships and planes that transport cable materials, resulting in lower greenhouse gas emissions (Schoon).
Recycling
At the current rate of metal extraction in ore mining, elements such as silver and cadmium are expected to be used up in the next fifty years (Awasthi et al). Recent research regarding e-waste recycling presents a potential solution to this problem since e-waste could be used as a secondary resource for many valuable metals. For example, the Environmental Protection Agency reported that 1 million mobile phones could provide about 9 kilograms of palladium, 24 kilograms of gold, 250 kilograms of silver, and over 9 tonnes of copper (Gopikrishnan et al). Despite the amount of precious metals in e-waste that could be refined for reuse, only around 20% of the e-waste is officially collected and recycled (“UN Report: Time”).
Recycling Methods
Bioleaching
Compared to other formal recycling methods, bioleaching, or biometallurgy, is among the most sustainable and environmentally friendly, however, its usage is currently limited to research laboratories and the mining industry (see fig. 2). The process of biometallurgy involves the use of microbial oxidation, where microbes oxidize inorganic compounds, and the microorganisms’ ability to directly or indirectly dissolve metals (Boon et al). Direct bacterial leaching requires several enzymatically catalyzed steps to increase the rate at which microbes bind with the e-waste base and oxidize the metals. However, In indirect bacterial leaching, instead of directly contacting the waste, the microorganisms produce a lixiviant, which is a liquid used in metallurgy, to oxidize and enhance the solubility of desired metals (Arya and Kumar). While hydrometallurgy and pyrometallurgy require high energy input and additional procedures, such as post-treatment and management of acidic wastewater, bioleaching has fewer requirements as its main objective is to support the microorganisms. Biometallurgy also produces the lowest level of pollution compared to other methods (Arya and Kumar). For example, from research conducted at the National University of Singapore, Yen-Peng Ting, an environmental chemical engineer, worked with Chromobacterium violaceum, which is a bacterium that produces an enzyme that converts glycine to hydrogen cyanide for indirect gold bioleaching. Through research, he and his colleagues found that the bacterium could convert excess cyanide to β-cyanoalanine, a nontoxic amino acid that the bacterium uses for growth and development (Kwok).
Bioleaching still requires deeper research as it is still neither as efficient nor as effective as other recycling processes. Foremost, chemical leaching usually extracts metals at a faster rate than bioleaching (Pant et al). Unlike biometallurgy, most recycling methods also recover nearly 100% of the desired metal. For example, through the many experiments Ting and his colleagues conducted, C. violaceum recovered a maximum of 69% of the gold in e-waste (Kwok). Thus, much more research is needed to identify innovative methods of enhancing the activities of bioleaching microbes. Ting stated that bacteria-produced cyanide should be one of the main focuses when researching biometallurgy (Kwok). However, to allow further research, more funding and collaborators would be required.
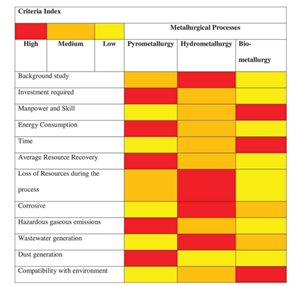
Fig. 2. Comparative Assessment of Treatment Options for Resource Recovery from E-Waste from Priya and Hait; doi.org, 14 June 2020, https://doi.org/10.1080/21655979.2020.1775988.
Plastic-eating Bacterium
After extracting valuable substances for reuse, eliminating the leftover plastic is crucial since the toxins are damaging to the environment and human health. One way to reduce the amount of plastic is bio-recycling, specifically, relying on a plastic-eating bacterium named Ideonella sakaiensis. This bacteria consumes PET plastic, or polyethylene terephthalate, a material used in electronics because of its insulating properties (“What is PET”). I. sakaiensis degrades this plastic through its secretion, and the PET consumed acts as the bacterium’s main energy source (see fig. 3). With a tolerance range that is easy to achieve, cultivating the bacteria to consume the plastic would be attainable (“Ideonella Sakaiensis”). However, using this bacterium is not the most effective method of recycling plastic, and although it is more efficient than many other forms of bio-recycling, more research could drastically increase its productivity (Flashman).
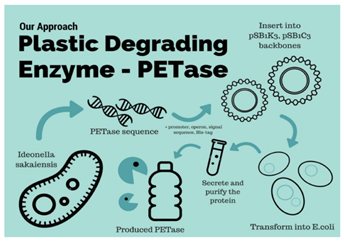
Fig. 3. Ideonella sakaiensis plastic breakdown process; UoA New Zealand; 2016iGem.com, http://2016.igem.org/Team:UoA_NewZealand/Project.
Conclusion
Given the immense amount of pollutants produced from e-waste and its extreme effect on both the environment and human health, it is crucial to reduce the production rate of e-waste and to minimize its harm as soon as possible. Many aspects of the electronics industry, including the transparency of materials, product packaging, and the production and recycling processes, should be improved to lessen the impact of e-waste. Throughout the development of potential solutions, it is especially important to stay mindful of how society’s decisions and actions contribute to the increase of e-waste.
Works Cited
Arya, Sashi, and Sunil Kumar. “Bioleaching: Urban Mining Option to Curb the Menace of E-
Waste Challenge.” Taylor & Francis, 14 June 2020,
www.tandfonline.com/doi/full/10.1080/21655979.2020.1775988. Accessed 30 July 2020.
Awasthi, Abhishek Kumar, et al. “Environmentally Sound System for E-Waste:
Biotechnological Perspectives.” Current Research in Biotechnology, Elsevier, 28 Oct.
2019, www.sciencedirect.com/science/article/pii/S2590262819300097. Accessed 30 July
2020.
Bohn, Dieter. “Apple Might Not Include a Charger with the IPhone 12. Good.” IPhone 12:
Why Apple May Not Include a Charger - The Verge, The Verge, 30 June 2020,
er-charger-rumor-usb-c. Accessed 30 July 2020.
Boon, M., et al. “The Mechanism and Kinetics of Bioleaching Sulphide Minerals.” Taylor &
Francis, 28 Nov. 2010, www.tandfonline.com/doi/abs/10.1080/08827509608962433.
Accessed 30 July 2020.
Cho, Renee. “What Can We Do About the Growing E-Waste Problem?” State of the Planet,
27 Aug. 2018, blogs.ei.columbia.edu/2018/08/27/growing-e-waste-problem/. Accessed
30 July 2020.
Duan, Huabo, et al. “Characterization and Inventory of PCDD/Fs and PBDD/Fs Emissions
from the Incineration of Waste Printed Circuit Board.” Environmental Science &
Technology, 28 June 2011, pubs.acs.org/doi/abs/10.1021/es2007403. Accessed 30 July
2020.
Flashman, Emily. “How Plastic-Eating Bacteria Actually Work – a Chemist Explains.” The
Conversation, 12 Sept. 2019, th econversation.com/how-plastic-eating-bacteria-actually-
work-a-chemist-explains-9 5233. Accessed 30 July 2020.
González, Alejandro, and Pauline Overeem. “Beyond Corporate Transparency.”
GoodElectronics, 14 Apr. 2020, goodelectronics.org/beyond-corporate-transparency/.
Gopikrishnan, V., et al. “Microbial Leaching of Heavy Metals from e-Waste: Opportunities
and Challenges.” Biovalorisation of Wastes to Renewable Chemicals and Biofuels,
Elsevier, 28 Feb. 2020,
www.sciencedirect.com/science/article/pii/B9780128179512000109. Accessed 30 July
2020.
“Harmful Effects.” GreenCitizen, 29 July 2019, greencitizen.com/learn-more/harmful-effects/.
Accessed 30 July 2020.
Kwok, Roberta. “Inner Workings: How Bacteria Could Help Recycle Electronic Waste.”
PNAS, National Academy of Sciences, 15 Jan. 2019, www.pnas.org/content/116/3/711.
Accessed 30 July 2020.
Loughran, Jack. “E-Waste Quantities Booming While Recycling Rates Decline.” RSS, 3 July
recyclingrates-decline/. Accessed 30 July 2020.
Nimpuno, Nardano, et al. “Information on Chemicals in Electronic Products.” Diva-Portal,
Norden, 2011, www.diva-portal.org/smash/get/diva2:702629/FULLTEXT01.pdf. Accessed
30 July 2020.
Pant, Deepak, et al. “Chemical and Biological Extraction of Metals Present in E Waste: A
Hybrid Technology.” Waste Management, Pergamon, 2 Jan. 2012,
www.sciencedirect.com/science/article/abs/pii/S0956053X11005423. Accessed 30 July
2020.
Peters, Jay. “Samsung Reportedly Won't Include Chargers with Some Phones Starting next
Year.” The Verge, The Verge, 8 July 2020,
ironment. Accessed 30 July 2020.
“Polyethylene Terephthalate (PET): A Comprehensive Review.” Omnexus, Omnexus,
Accessed 30 July 2020.
“PVC: The Poison Plastic.” PVC: The Poison Plastic, 18 Aug. 2003,
e-poison-plastic.html. Accessed 30 July 2020.
Reuters, Thomson. “Record 53.6 Million Tonnes of e-Waste Dumped Globally Last Year, Says
UN Report | CBC News.” CBCnews, CBC/Radio Canada, 2 July 2020,
www.cbc.ca/news/technology/global-ewaste-monitor-2020-1.5634759. Accessed 30 July
2020.
Schoon, Ben. “Samsung May Follow Apple in Ditching Included Charger - 9to5Google.”
included-report/. Accessed 30 July 2020.
Semuels, Alana. “Electronic Waste Is Becoming a Global Environmental Problem.” Time,
Time, 23 May 2019, time.com/5594380/world-electronic-waste-problem/. Accessed 30
July 2020.
“The Environmental Push for Ethical Electronics Manufacturing.” Great Lakes Electronics, 9
electronicsmanufacturing-be-the-solution/. Accessed 30 July 2020.
“UN Report: Time to Seize Opportunity, Tackle Challenge of e-Waste.” UNEP - UN
Environment Programme, 24 Jan. 2019, www.unenvironment.org/news-and-
Accessed 30 July 2020.
Wikipedia contributors. “Brominated Flame Retardant.” Wikipedia, Wikimedia Foundation,
13 Apr. 2020, en.wikipedia.org/wiki/Brominated_flame_retardant. Accessed 30 July
2020.
Wikipedia contributors. “Ideonella Sakaiensis.” Wikipedia, Wikimedia Foundation, 4 Aug.
2020, en.wikipedia.org/wiki/Ideonella_sakaiensis. Accessed 30 July 2020.
World Bank Group. “Electronics Manufacturing.” Environmental-Expert, 1 Jan. 1998,
d3pcsg2wjq9izr.cloudfront.net/files/8509/articles/1381/57_elect.pdf. Accessed 30 July
2020.
Comments